In 2001 D.R. Liu and co-workers at Harvard University employed the ability of a DNA-double helix to direct region-specific chemical reactions to the construction of DNA-encoded chemical libraries.
By bringing two DNA-linked reagents (termed “template” and “reagent”) in close proximity through Watson-Crick base pairing (“proximity effect”), the local increase of reaction molarity allows the expected chemical reaction to occur, even at nominal concentrations that are several orders of magnitude lower than conventional solution-phase reaction. As such “proximity effect” is nearly constant within a distance of 30 nucleotides, various architectures featuring multiple reagent-coding sequences at different positions on the same DNA-template can be conceived (Figure 10).
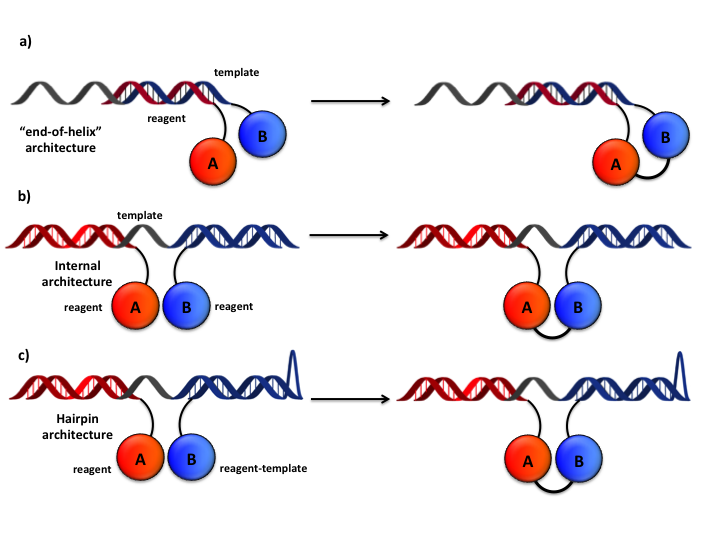
Figure 10: DNA-templated architectures. a) “End-of-helix”: the two reagents are displayed on 5’ and 3’ extremities of two complementary oligonucleotide sequences. b) “Internal” setup: the displayed reagents are confined in a limited reaction space by means of a longer oligonucleotide “template” carrying both complementary sequences. c) “hairpin” configuration: a longer oligonucleotide serve as both “reagent” and “template” oligonucleotide.
To date, such DNA-directed reactions have been employed to the construction of DNA-templated libraries and to the development of the Yoctoreactor technology:
DNA Templated Libraries
Adopting an “end-of-helix” setup (see Figure 10a) for the DTS (DNA-Templated Synthesis) assembly, in 2008 the group of Liu presented the construction of a macrocyclic fumaramide DNA-encoded library containing 13’824 compounds, starting from 8 different initial “templates” and using in total 36 “reagent” building blocks [(12 x 12 x 12) x 8] (Figure 11).
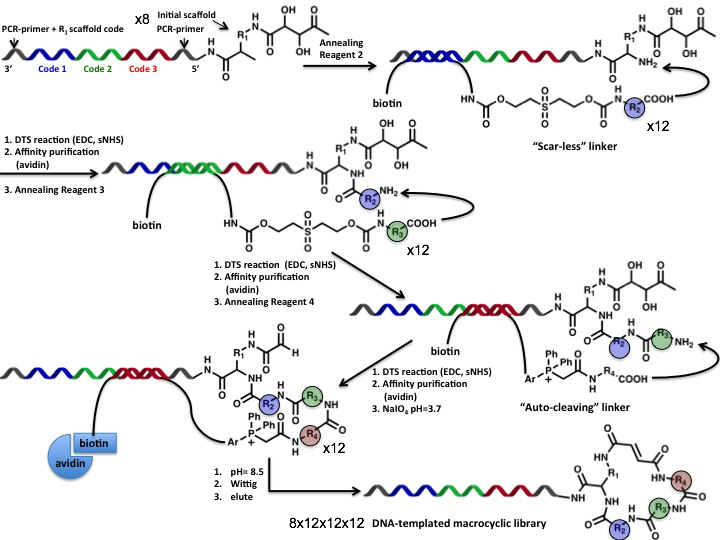
Figure 11: A discrete number of DNA-linked scaffolds simultaneously undergo three consecutive DNA-templated reactions using different DNA-templated reagents. Conjugate intermediates are purified by affinity capture on avidin/streptavidin resin and elution in denaturing condition. Scar-less linkers (i.e., base labile sulfone linkers) allow introducing suitable functional groups for the next reaction step, while auto-cleaving linkers are typically used to trigger the final macrocyclic ring-closure reaction (i.e.,by means of Wittig reaction).
The library as described in Figure 11 has been used for direct in vitro selection against numerous target
proteins, enabling the identification of various kinase inhibitors and activators. In analogy with “DNA-routed” libraries, the DNA-tag serves both for encoding and for programming the library synthesis. However, for “DNA-templated” libraries, the “molecular evolutionary process” has not been experimentally demonstrated.
As at low concentration DTS-reactions uniquely depend on reagent/template annealing, each DNA-pair behaves as independent “molecular reactor”. Therefore, only complementary DNA-reactants yield to intra-molecular products. This principle has been applied by Liu and co-workers for the high-throughput discovery of new chemical reactions. In brief, two pools of DNA-linked substrates were mixed at minimal concentrations (nM) and simultaneously evaluated fo r bond-forming reactions. The setup allowed the discovery of a novel Pd(II)-mediated carbon–carbon bond reaction between a terminal alkyne and a mono-sobstituted alkene as schematically represented in Figure 12. Interestingly, the new DNA-templated reaction also operated in non-DNA-templated format.
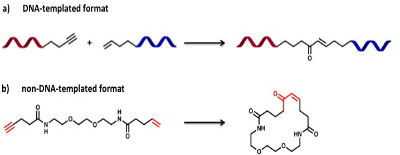
Figure 12: new alkyne–alkene macrocyclization reaction by DTS. a) DNA-templated format, the reaction was performed incubating the reagents with 500 mM Na2PdCl4 at 37°C for 1 h, or at 25°C for 20 min. b) non-DNA-templated format, the reaction performed in similar conditions yielded the expected macrocyclic enone product. The reaction probably proceeds through the following steps: formation of Pd(II)–alkynyl intermediate; insertion of the alkene into the Pd-alkyne bond; beta-hydride elimination to form a conjugated enyne; Pd(II)-mediated hydration of the alkyne to form an enol pi-allyl Pd-complex; tautomerization and pi-allyl Pd protonation to generate the final trans-enone.
In the “zipper-box”(Figure 13), the DTS-reaction between two DNA-reagents consecutively annealed on the same single-stranded DNA-template is modulated controlling the association/dissociation of two adjacent DNA-conjugate reactants (e.g., performing PCR-like thermal cycles or using appropriate high/low salt concentration buffers).
If a bi-functional DNA-linked moiety is included, the iteration of the process allows multiple one-pot DNA-templated reactions to take place on a single DNA-linked core structure (Figure 13).
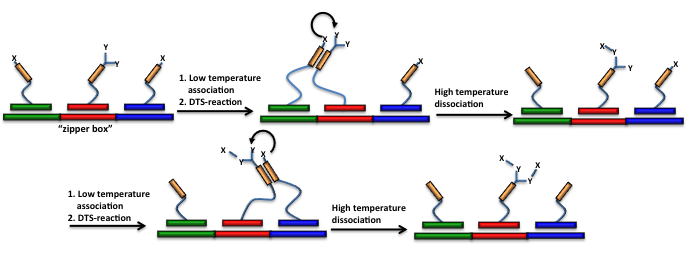
Figure 13: “Zipper box”: DNA-conjugate reagents, each containing two hybridization domains, are consecutively annealed on on the same single-stranded DNA-template. Association/dissociation of two adjacent DNA-conjugate reagents triggers the DTS reactions. If a bi-functional DNA-linked moiety is included, association/dissociation cycle can be iterated enabling multiple one-pot DNA-templated reactions to occur on the initial DNA-template.
Yoctoreactor technology
The Yoctoreactor (yR) approach (Figure 14) exploits the geometry and the stability of a three-dimensional structure – a DNA-junction – to create chemical reactors each capable of synthesizing and encoding a unique compound. First, chemical building blocks (BBs) are coupled to bispecific DNA oligonucleotides (oligos) using cleavable or non-cleavable linkers. The oligos comprise the DNA-barcode for the attached BB as well as structural elements of the reactor. The bispecificity of the oligos lends to spontaneous combinatorial assembly into the DNA junction. The building blocks are positioned on the oligos such that upon assembly they are confined at the center of the DNA junction within a volume on the order of yoctoliter (10-24 liter). By virtue of the “proximity effect”, chemical reactions between BBs take place at the center of the chemical reactor – the 3D nature of the reactor having a favorable effect on the efficiency of the reactions.
YoctoReactor libraries can be assembled in either of two ways:
a) stepwise, leading to linear and branched compounds (Figure 14a).
b) in a single step using multi-component reactions leading to scaffolded compounds (Figure 14b).
After the chemical reaction the products are purified, thus eliminating any unreacted species. The oligoes are ligated to form a continuous strand of DNA encoding the chemical product, then all linkers between the DNA and the chemical product except one are cleaved. Library formation is finalized by a single PCR cycle (primer extension) to yield a library of small molecule compounds attached to double stranded DNA at a single point. Library size and diversity is controlled by the geometry of the YoctoReactor used and number of different BB-linked oligos used in each position.
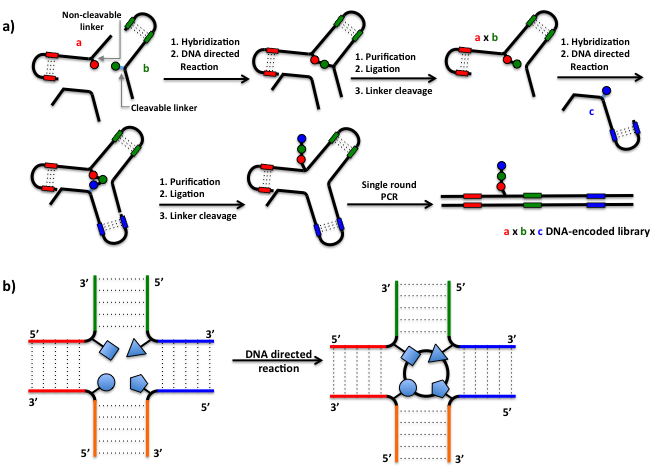
Figure 14: The yR approach exploits the geometry and the stability of a three-dimensional DNA-junction to generate chemical reactors capable of simultaneously synthesizing and encoding a unique library member compound. a) yR stepwise assembling: stepwiseDNA-directed reactions yield a library of small molecule compounds attached to double stranded DNA at a single point. Library size and diversity is controlled by the geometry of the YoctoReactor used and number of different BB-linked oligos used in each position. b) yR single-step assembling: simultaneous multi-component DNA-directed reactions lead to one-pot synthesis of a scaffolded DNA-encoded compound library. Library synthesis is completed cleaving all linkers between the DNA and the chemical product except one, and performing a single PCR cycle to dismantle the molecular reactor and displaying the final small-molecule library on linear double-stranded DNA templates (see Figure 14a).